Biochemical Wearables, Ingestibles, Implantables: In Between a FitBit and CAR-T Therapy
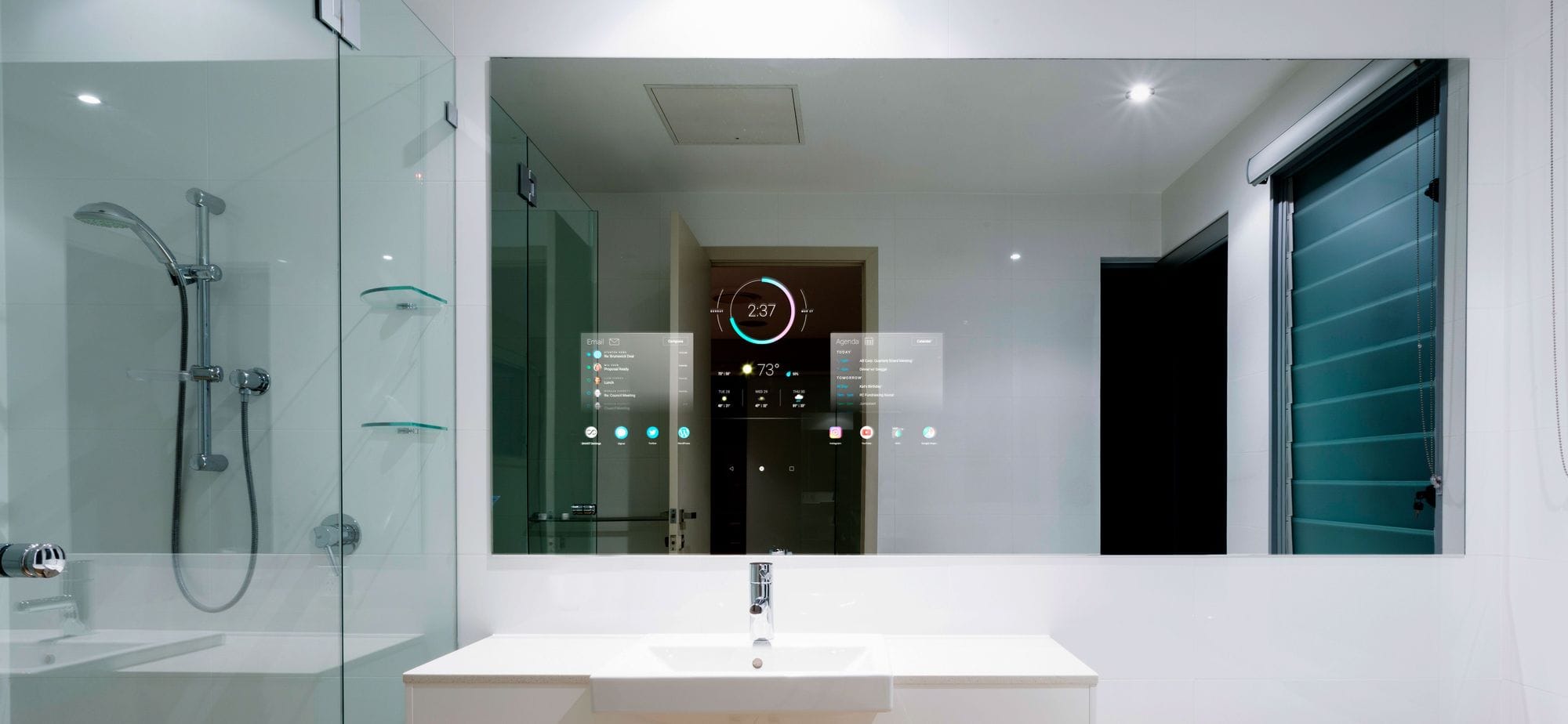
Innovation outside of pharmaceuticals has gone relatively underappreciated by biotech VCs and the market. In fact, the single greatest biotech success story for a startup founded over the last several decades has nothing to do with therapeutics. That honor goes to Intuitive Surgical which developed surgical robotics all the way to a $200B valuation – 12x that of Moderna.
We at Compound are excited about new waves of innovation across medical devices that have the potential to transform healthcare and how it’s delivered, from non-invasively measuring our body’s biochemistries at the molecular level to microrobotics navigating inside the body to non-pharmaceutical modulation.
Noninvasive measurement of health and disease at the molecular level
The first generation of wearables and continuous health monitoring devices ranging from FitBit to Apple Watch to 8 Sleep use light, motion, and pressure sensors to track a small subset of health indicators – namely heart rate, temperature, blood pressure, blood oxygen, and body movement.
The arrival of continuous glucose monitors after decades and billions in R&D heralds a fundamentally new era: biochemical wearables. These enable for the first time an interface between electronics and the body’s biology at the level of individual biomolecules. They quantify the hormones, cytokines, ions, metabolites, drugs and nutrients circulating in our blood that make their way into ISF (and to a lesser extent sweat) by turning the binding event of enzyme-, antibody- or aptamer-based sensors into an electrical or optical signal.
They’re like noninvasive, continuous blood tests. Consider how diabetes management has been revolutionized. Until recently, the standard of care was doing blood tests and injecting oneself with a needle full of insulin a handful of times a day. Now it’s a fully closed-loop, minimally invasive system where the CGM continually tracks blood sugar with barely any physical discomfort and wirelessly communicates with the user’s insulin pump to autonomously infuse the exact right dose in real time. Abbot, Dexcom, and Medtronic are commercializing the tech to the tune of tens of billions in revenue a year.
However, CGMs are anything but a general-purpose technology platform. CGMs benefited from the facts that glucose is a relatively large and highly abundant molecule, and glucose happens to be the only relevant target with a naturally occurring enzyme that oxidizes it, a process that innately produces an electrical current. The main R&D tricks were getting the system to be stable for weeks at a time and miniaturizing everything.
I’ve heard the medtech giants are well behind the cutting edge academic labs in developing more generalized biochemical platforms as they’re focused on commercializing the golden goose they have. With that said, they’d likely make for great acquirers given their expertise in manufacturing and distribution – a path analogous to the biotech to pharma pipeline.
The cutting edge academic labs’ tech utilizes molecular switches like aptamers and antibodies. These biological fragments can be selected for their binding affinity to a given target and can often be purchased wholesale from vendors like SomaLogic. The difficulty has been getting them to change structure in a predictable way, creating sufficiently sensitive electrodes or optics and standardized chemical processes to stabilize them for long enough in vivo use.
Excitingly, that work has matured to the point of being spun out into startups that are working towards clinical trials. With sensor multiplexing, we’re nearing the ability to measure many different biomolecules simultaneously and continuously at picomolar sensitivity.
Near-term business models range include:
- Partnering with hospitals for out-patient monitoring of major diseases. Many examples like heart congestion have billions in economic cost annually with no effective way to remotely monitor patients for disease recurrence.
- Continual monitoring of chronic diseases like female hormone monitoring for menopause or reproductive management, stress quantification, gout, systemic inflammation
- D2C, non-reimbursable sales for fitness and nutrition optimization (amino acids, electrolytes, lactate). While working out makes sweat sensors easier because it doesn’t require sweat stimulation or FDA approval and so several companies have already popped up, we’re least excited by this because it has the weakest need and least favorable economics.
- Partnering with drug developers to provide real-time tracking of their drug’s pharmacokinetics in each patient’s blood stream. For many drugs like chemotherapy or pediatrics with steep dose response curves, narrow therapeutic windows, and/or unpredictable dose-blood concentration relationships, getting the exact dosing correct for each patient is a long-standing challenge and causes many such drugs to fail in late development stages. Dosing is currently calculated based on the patient’s weight and/or height, not accounting for differences in how peoples’ bodies metabolize drugs, how much is absorbed into the bloodstream, etc. With the help of biochemical wearables, implantables, or IVs, the clinician sets a priori the desired concentration profile over time and then the system delivers exactly that for every patient based on real-time blood concentration levels.
Like CGMs, these will start as medical devices for specific serious conditions but may eventually be used routinely by consumers if the price comes down from $100s-$1,000s, sensor stability extends beyond a couple weeks, and as biohacking becomes more prominent.
Additionally, the consumer market and exit opportunities for companies may expand if robust, continuous wearable sweat sensors can be developed. Researchers I talked to were highly skeptical of their prospects for real world deployment. However, if their challenges are eventually overcome, then they’d offer an even less invasive and more consumer friendly option.
Whoever successfully expands to the consumer market or has a clear path towards it unlocks not only a larger install base but also possibly exit opportunities to consumer tech companies like Apple and Samsung as opposed to strictly medtech giants.
Another significant unlock would be adding the capacity to actively respond to disease flare ups by administering the requisite drug in corresponding doses in real time. The sensors often use microneedle patches, which are clinically validated for vaccine delivery, so maybe some portion of the array unused for sensing could be used for drug delivery.
Some day, biochemical sensors will come in other form factors beyond wearables and be embedded more deeply and passively into daily life. A company made a smart tampon but samples needed to be sent in the mail to the lab; maybe a future approach could analyze it in situ.
Meanwhile, researchers and startups are taking smart toilets beyond bidets to mine stool and urine for relevant data. First-generation integrated smart toilets strictly apply visual analysis to track color, pressure and size as on-site biochemical or genetic sequencing isn’t currently practical.
However, startup BiomeSense developed an innovative work-around by building a lab-in-a-box that sits next to the toilet that automatically processes used toilet paper to extract the microbiome (very much not shelf-stable which drives errors in data in traditional kit-based, one-off stool collection) and store its DNA (extremely shelf-stable) so it can be sent into centralized sequencers just once a month.
Most importantly, they paired that solution to the otherwise taboo, low-adherence sample collection with a clever GTM by selling to clinical trial operators, yielding big contracts (as such a device will long be expensive for DTC) and helping the researchers publish their papers they wouldn’t otherwise be able to while BiomeSense gets rich metadata on not just the patient’s daily microbiome but their full EHR. In the longer term, maybe this can fund an integrated toilet that does on-site, continual, passive sequencing once Oxford Nanopore gets cheap enough. This thoughtfulness to GTM and business model is equally rare and essential in this field.
The even longer-term vision for embedding powerful biomolecular screening into the background of life was perfectly encapsulated by the CEO of Oxford Nanopore who envisions a miniaturized DNA sequencer inside your toothbrush, serving as a twice-a-day liquid biopsy scanning for cancer, microbiome imbalances, etc:
An ideal device would be a toothbrush, I think. There's no reason why we can't have a small integrated sequencer that would fit inside a toothbrush. And when you clean your teeth, if you clean them well, there should be a little bit of blood. You're basically opening up cells and releasing the DNA. In the mouth you have 50,000 white blood cells per mL. When you scrape your teeth, you release around 100mL of serum or blood. Doing that twice a day if you sum over the stuff you are measuring, you're looking at 10, 20mL of blood, in tiny steps. We can select molecules, filter molecules, if we see a molecule that looks suspicious, we can sequence it again and again and be certain that we're seeing a pathogenic mutation. That all sounds completely bonkers but that's where I want to take this technology over the next 20, 30 years.
Adding biochemical sensors to traditional electronic wearables and health devices will be like moving from continuous vitals measurements to continuous full-body concierge-doctor quality check ups. Eventually, they will be perennially scanning for and managing complex disease states in the background of normal life.
Traveling Withinside the Body to Sense Disease and Deliver Drugs
Some things can only be measured and acted upon from withinside the body, which requires even more dramatic miniaturization of the sensing and energetic components than wearables. A range of implantable and ingestibles devices are on the horizon that are moving the field beyond sensing basic parameters like pressure or temperature, a parallel development to the section above. These devices have increasingly complex actuations like responding to specific parameters by releasing the drug load or collecting samples from the body while others have invented ways to deliver drugs that otherwise couldn’t be delivered.
Ingestibles, historically pill-shaped inflammation sensors for GI issues, are being developed for active microbiome sampling. These untethered, standalone robotic capsules sense the change in the pH upon leaving the stomach, then collect samples from the intensine’s inner wall by either mechanically opening and closing or by using passive techniques, and fully seal to store the sample without contamination on its journey out of the body for analysis in the lab. Maybe future versions will be able to collect the biochemical data internally without requiring the patient to fish through their toilet.

Even more miniaturized robotic devices have been developed to travel through far narrower pathways than the gut to deliver drugs to regions of the body otherwise unreachable. Prevailing drug delivery methods are systemic, diffusing throughout the entire body and increasing odds of off target effects. Even with recent advancements towards tissue-specific targeting with nanoparticles and viral vectors, some areas of the body may long be out of reach for biological carriers.
A prime example of this paradigm is Compound portfolio company Bionaut which uses external magnetic propulsion to navigate up the spine or through the brain’s reservoirs of cerebrospinal fluid to the brain stem where it delivers its modality agnostic payload. Designed in a variety of shapes from screws to cylinders, they can move through simple Newtonian fluids to tumors and fibrous tissue.
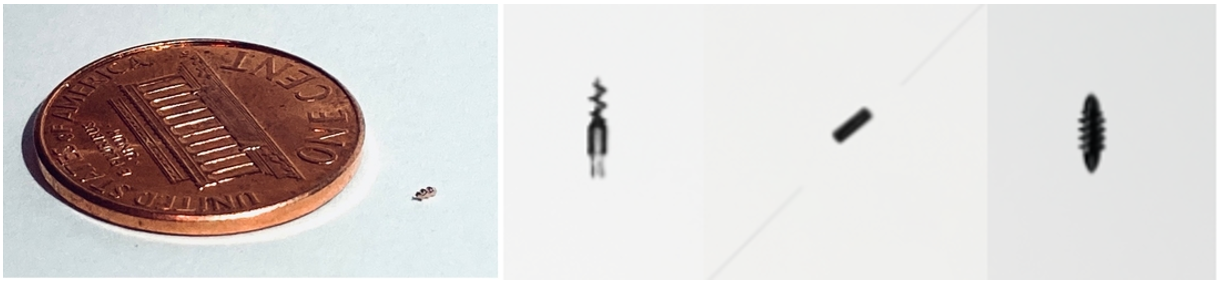
Finally, implantables have moved from passively sensing basic health parameters to actively responding to flare ups in real-time by safely housing the required drugs and releasing them in the precise dose called for. The ultimate goal for these devices is to enable closed-loop therapy for managing chronic diseases from cancer to diabetes. Or, as a leader in the field said, “it’s like an implantable pharmacy on a chip that never runs out.”
For many such chronic diseases, the end state of medical intervention may be cell therapy, which can verge on true cures. However, injected cells often die of oxygen deprivation and the immune system rejects foreign cells, requiring continual immunosuppressive drugs for even autologous cell therapies.
For diabetes, MIT engineers developed a bioelectronic device that houses insulin-producing pancreatic islet cells, protecting them from the immune system and feeding them oxygen. Magnetic forces from outside the body power its onboard oxygen generator that indefinitely splits water vapor found in the body into oxygen and hydrogen via a proton-exchange membrane. In mice, the protected islet cells responded properly to surges in blood glucose levels. Such a device could possibly be adopted for other diseases requiring continual delivery of proteins.
A well-funded ARPA-H moonshot aims to do a similar thing for immunotherapy delivery for patients of hard to treat cancers like ovarian and pancreatic. The group created a 1cm implant to house living cells that would both synthesize and deliver the therapy as needed. “Instead of tethering patients to hospital beds, IV bags and external monitors, we’ll use a minimally invasive procedure to implant a small device that continuously monitors their cancer and adjusts their immunotherapy dose in real time.” The same group also leads a DARPA project to develop a fully implantable device hosting cells that produce the same peptides that regulate sleep cycles, with dose and timing controlled by light from the device. These projects aim to reach clinical trials in five years.
In the longer term, implantables will work towards better localized targeting with longer engrafting, lessened bodily rejection via the immune system’s fibrotic response, and long-lasting power. In the last several years, breakthroughs in powering the devices with wireless, external sources like electromagnetic energy enabled their miniaturization, as the battery is the largest component. A further inflection point would be the successful development of technologies to harness the various forms of energy abundant in the body for fully self-renewing power and locomotion. An exciting recent proof of concept demonstrated an implantable battery that runs on the body's oxygen and sodium and here’s a review on all possible approaches. Such advances would enable very long-term implantation and thus the closest thing to cures for chronic diseases.
Another far-out development would be the ability for the devices to implant themselves in the targeted tissue, alleviating the need for any form of operation and thus making them fully non-invasive. Maybe a surgeon’s external mechanical force will always be necessary to stably implant a device inside the body, but clever materials science advances have shown glimmers of a more sci-fi future, including untethered 2mm-wide soft robots that navigate complex vasculatures to remove blood clots with magnetic propulsion strong enough to swim against the bloodstream as well as soft robotic grippers that autonomously wrap around and conform to body parts to act as electric implants. Who knows, if mechanical force can be addressed, maybe microrobotic surgeries can unlock treatments like solid tumor biopsies as uninvasive as checkups.
Non-pharmaceutical modulation
Devices to measure and modulate the body’s circuitry with electricity or ultrasound instead of traditional pharmaceuticals have exploded over the last decade. The technological frontier moved from the first pill-sized pacemakers in 2016 to implanted brain-chip interfaces enabling paralyzed patients to navigate computers with their mind and maybe even curing forms of blindness.
BCIs have benefited not just from miniaturization of electronics, power sources, and more biocompatible materials but also an exponential rise in electrode density and with it a corresponding increase in data collected per square inch. They’ve now reached 0.1-1mm brain tissue resolution.
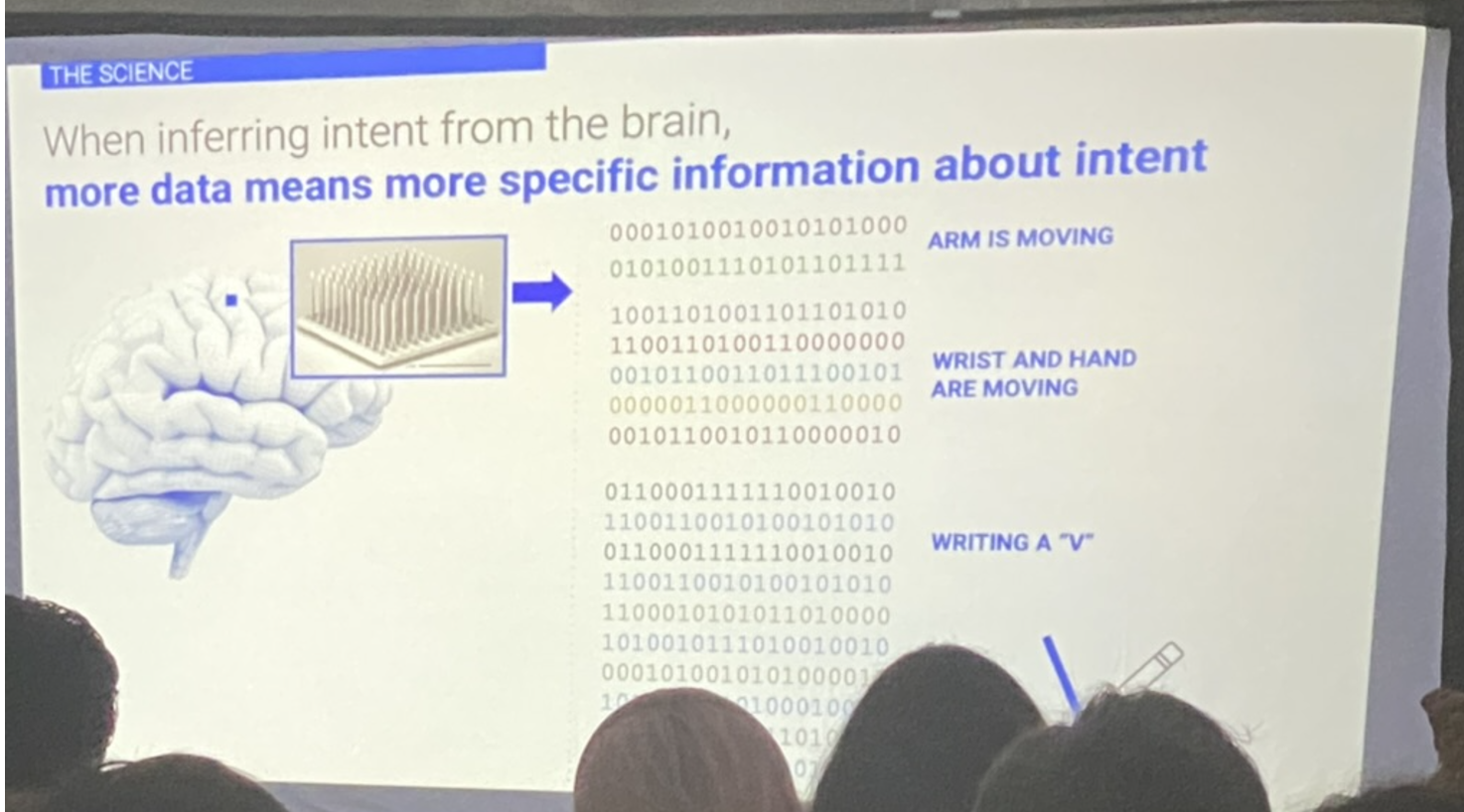
With implanted BCIs’ success increasingly established, innovation has shifted towards minimizing invasiveness to figure out how to retain useful capabilities without the need for surgery. For instance, one of our stealth portfolio companies is creating a non-invasive consumer-oriented device.
Other groups are experimenting with alternative mediums for measuring brain activity beyond electricity like miniaturized fMRI machines which can measure whole-brain activity instead of the neurons directly hooked up to the implanted electrode. In this vein, researchers recently demonstrated reconstructing the images that people are imaging in their mind.
The next step is to go from collecting data from the brain to modulating it, from reading the brain to writing to it. Implantable devices that electrically stimulate the central or peripheral nervous system are now used to treat psychiatric and pain disorders and for movement disorders like Alzheimer’s or spinal cord injuries.
Meanwhile, noninvasive EEG consumer products have popped up everywhere in the last couple years from headsets to help ADHD patients’ neurons reach full action potential to stimulation for sleep inducement. While time will tell how effective these current generation consumer EEG headsets are, other groups targeting non-invasive neuromodulation are turning to focused ultrasound (FUS) which has enjoyed repeated demonstrations of safely controlling various regions of the brain. Several startups have emerged to commercialize the breakthrough.
In the longer term, the field may achieve single-neuron specificity. IN-BRAIN is developing graphene FETs with such a promise, though only for neurons on the brain’s surface. Meanwhile, cell membrane anchors, magnetogenetics, magnetorestrictive particles may provide non-invasive control of neural circuits anywhere in the brain at cellular specificity, but the fields are still in basic science development and safe delivery must be achieved for it to be a viable therapeutic approach for humans and thus would likely only become viable once Alzheimer’s pharmaceuticals become fully de-risked.
Whether it be EEG, fMRI, FUS or magnetogenetics and implantable chips, non-invasive skin-adhering devices or headsets, whoever truly unlocks decoding the brain will benefit those with acute disorders from paraplegics to population-scale diseases like Alzheimer’s.
As minimally invasive devices improve in utility and social customs normalize their usage, BCIs will also go beyond treating disease to boosting the healthy population’s understanding of their own thoughts, moods, and mental patterns, and even modulate them for the better.
Crucially for industry dynamics, exponentially more data fuels step functions gains in capabilities, accuracy, and expressivity for these systems, a dynamic less relevant for the other devices mentioned throughout this post. Indeed, researchers have started teasing out the scaling laws for EEG neural data. This means that the self-reinforcing, winner-take-most dynamics in AI will likely play out with these devices such that whoever collects the most data will be able to deliver the most useful insights.
We at Compound are especially excited about these approaches that can collect large streams of data to feed into AI models to then improve the product for all users as well as possibly use that data as a platform to expand into new services or to go from reading the brain to writing to it.
For instance, companies could prove out their technology for reading brain activity to analyze mental patterns and moods, use that as a way to offer therapy that understands your mental activity better than you do (at the level of neuronal firing), build the industry-leading dataset, and then use that data to create neuromodulatory offerings.
Finally, unusually creative approaches to modulate the brain are efforts to do so via the gut, which has the second most neurons in the body outside the brain and is known as the “little brain.” New noninvasive ingestible devices navigate to the nerve endings in the GI tract that connect to the brain, connect their electrodes to the mucosa, and wicks away the stomach’s liquid with hydrophilic grooves. Clinical indications include chronic nausea and eating disorders like ARFID. Maybe they can join the GLP-1 hype train too.
Electroceuticals are being developed for many other parts of the body beyond the brain, providing electrical pulses when disorders cause under-stimulation as pacemakers provide proper regulation of the heart.
From Basic Parameters to Biochemical and from Passive to Active
The microelectronic devices discussed throughout have all benefited from the miniaturization of electronics and batteries combined with advances in their respective sensors and biocompatible interfaces. The first functional devices emerged in the 2000s and then miniaturized versions appeared in 2010s that had basic, passive functions. Now, devices are going from sensing a few basic parameters to sophisticated biochemical sensors, enabling non-invasive measurements that could previously only be collected via blood tests. Other devices are moving from passively sensing data to being able to respond to signals in real-time by delivering the precise therapeutic intervention called for by the sensed disease state, solutions that range from effective cures to chronic diseases to “writing” to the brain.
We’re extremely excited by these devices becoming even more powerful and less invasive over time with each minor scientific miracle and with each product iteration.
If you're building anything mentioned here across implantables, ingestibles, biochemical sensors or other novel types of next-generation medical devices we haven’t imagined, we'd love to chat