Dozens of Nobel-Worthy Innovations Awaiting Biomanufacturing 2.0
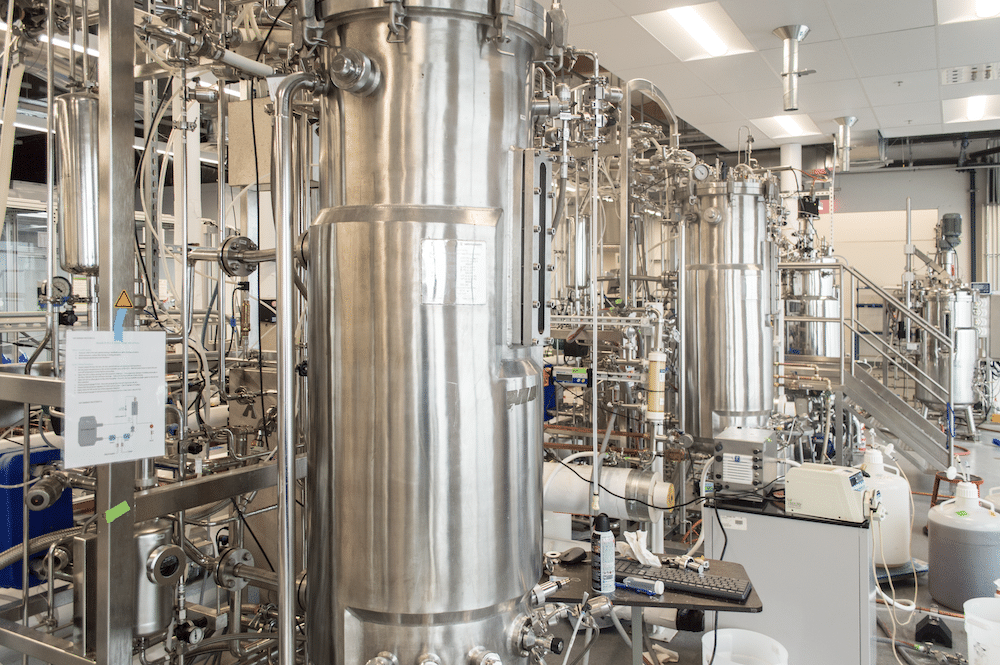
Many thoughtful breakdowns of Synbio 1.0 have pointed to poor product selection, the difficulty of addressing commodity markets on a venture timeframe and of retaining the data value from horizontal platforms. We at Compound feel each of those lessons ought to be internalized but that of equal if not greater importance is that the markets may be too fragmented at their early stages with companies commercializing just one somewhat incremental technology each.
In spite of widespread negativity, we remain steadfast in our belief that the diffusion of these technologies are a “when, not if” question. The field already has notched underappreciated proof-points and enjoys long-term tailwinds.
We’re excited about teams developing high-value products like science-driven consumer products or therapeutics as well as exceptionally strong teams taking on radical technical risk going after bulk products by integrating a handful of the 40+ Nobel-worthy technologies we detail below.
The Need for Radical Technical Risk, Especially for Commodity Products
Many startups centered their product selection on the world’s largest scale, lowest margin commodities, attracted by big TAMs and often impact. But the companies didn’t bring forth an equally transformative technological paradigm – one capable of a significantly lower cost structure than the large scale incumbents that have squeezed all efficiency out of the current paradigm.
Most first-gen companies commercialized one core piece of technology each. Impossible commercialized the biosynthesis of heme, cultivated meat companies a proprietary cell line, most biomanufacturing startups do either sterile or continuous or automated reactors.
But the tech is far too early for the commercialization of incremental or singular innovations. As recently as 2019, the production cost of lab-grown chicken was still $150/lb at best-case scenario, 200x the price chicken is sold for in B2B wholesale markets.
Even the more promising “Tesla approach” of first going after higher-end markets like blue-fin tuna or jet fuel or silk to get down the cost curve hasn’t exactly been easy. For instance, biomanufactured spider silk is still ~10x more expensive than normal silk.
The cost curves haven’t bent on a venture timeframe for mass production goods and so the most successful startups (e.g. Beyond, Impossible, Bolt, Amyris) struggled to turn a gross profit after tens of years and $100M-$1Bs invested. Forget about actual profit.
Indeed, in every podcast I’ve listened to with alternative meat leaders, when asked today whether it’s simply an engineering challenge that just needs to be scaled, none definitively said yes. Instead, they often pointed to the industry’s need for government support. While there are likely meaningful efficiencies to be had by solving engineering problems, it is equally or more likely that there’s still plenty of ambitious science to be done.
The Value Chain is Possibly Too Fragmented at the Industry’s Early Stage
Industries go through cycles of consolidation and fragmentation, but usually start with the former as bringing to market technologies and products that have never existed before requires end-to-end engineering. Once the product becomes standardized and commoditized, then the industry fragments into smaller suppliers specializing in one component.
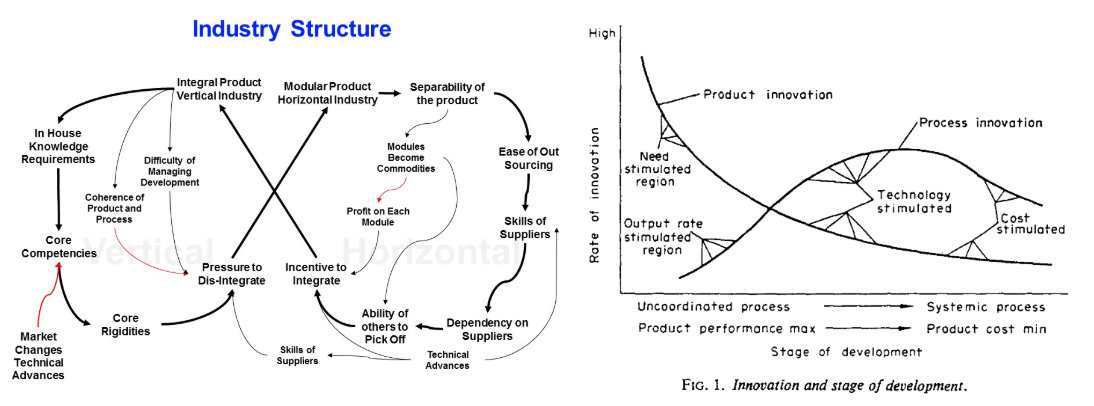
Biomanufacturing anything other than monoclonal antibodies seems too nascent an industry to be as fragmented as it is: suppliers specializing in cheaper growth factor manufacturing, others in the prediction of which of those growth factors to use with your cells, others in genetically editing your cells, others in growing your cells, and so on. Each must take their margin in an aggregate profit pool that’s… deeply negative.
Even more problematic for this market structure is that the demand pull isn’t there to overcome the cold start dilemma. We’ve talked with startups looking to commercialize growth factors that they believe at industrial scale could be 100x cheaper than FBS. But constructing those plants depends on huge demand for the end consumer product (e.g. cultivated chicken) that only materializes when it’s cheaper than what it’s replacing, which is only possible with the cheap growth factors. End demand for these products at premium prices isn’t there so incumbents and distributors rarely sign guaranteed offtake like we’re seeing in SAF, datacenters, and other industries with more demand pull.
With that said, maybe only incremental progress needs to be made such that the large startups of the 2015-2020 vintage that have raised massive sums will build their factories, get guaranteed offtake plus some material revenue so as to wean off the highest cost of capital in VC dollars to FOAK and then to project finance.
But that’s at least as risky a bet to make as taking on huge technical risk to verticalize the value chain yourself to come to market cheaper than incumbent products.
Looking Up from the Trough of Disillusionment
The actual wins that the industry has earned are just as underdiscussed as its failures are overdiscussed.
- Fuels: global biofuel capacity of 3M barrels with the largest biodiesel company generating $3B in revenue and $212M in profit, cargo ships’ demand exceeding supply, SAF production ramping exponentially with 10+ years of guaranteed offtake from major airlines
- Food: 8M L in global fermentation capacity, companies scaling sales like Eat Just having sold 500M egg equivalents, dozens of alt-meat companies that have started or planned 50-250K L plants, demos in fancy restaurants, products getting regulatory approval and hitting supermarkets, genetically modified crops silently taking over 100% of the market
- Pharmaceuticals: 17M L in global biomanufacturing capacity, engineered CAR-T cell therapies are one of the predominant waves
- Plastics & polymers: 2M tons of 100% bio-based goods produced a year with a current market size of $9-12B
- Fashion: the world’s largest maker of spandex fibers to spend $1B on a 200,000t plant that will use Genomatica’s fermentation tech, many small-volume lines and partnerships with top brands for startups like Spiber
- Chemicals: enzyme-based detergents rake in billions in revenue, Solugen and Pivot Bio are generating $100M in revenue; meanwhile, incumbents have started tinkering with the tech as BASF is deploying biocatalysts and Unilever invested $120m to scale ingredients alternative to seed-oil and fossil fuel cleansing products.
- Note that it’s possible the simplest commodity chemicals may not be ripe for disruption even with a Solugen-style bio-chemical hybrid approach as enzymatic pathways will always be longer and likely won’t outweigh their lower energy needs, as illustrated below. Instead, it’s likely the proper route to disrupting simple commodity chemicals is harnessing exponentially cheaper solar energy, especially by remodeling the process to be small-scale and intermittent friendly so as to remove the need for batteries entirely. Biomanufacturing should be used for more complex products.
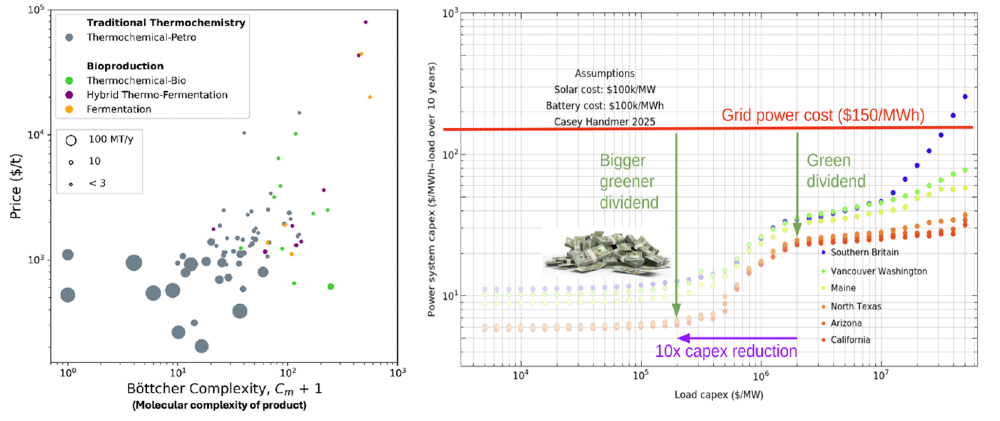
At this juncture, it’s worth remembering that it’s all still very early (e.g. 72% of all food fermentation investment ever occurred in 2021-23) and also that coming down the cost curve at scale has been done before with for example monoclonal antibodies (though it’s easier to start bootstrap that when the product sells initially for $200K per lbs).
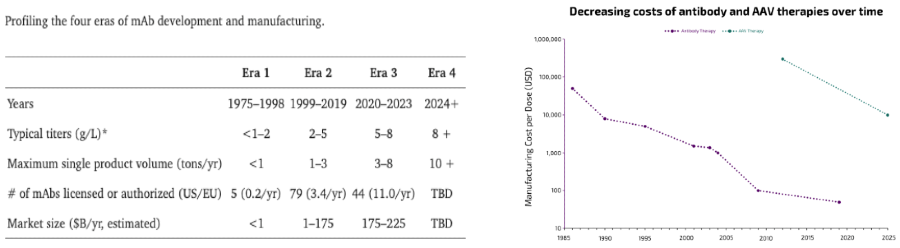
Moving forward, the premise of synthetic biology remains compelling as its applications are wide-ranging with large markets and the underlying tech continues to improve non-linearly.
Crucially, no theoretical biophysical limits restrict the tech from tackling applications outside of pharmaceuticals. And, the intuition remains that for example raising a 1,400lb cow to harvest a tiny portion of meat can’t be the most efficient technology possible.
It will take sustained investment over long periods with ambitious entrepreneurial attempts along the way. Encouragingly, there are now many public US institutions devoted to basic and applied research to pair with the strong entrepreneurial scene. Plus, many countries like China, Japan, Israel, and South Korea that are leaders in technological and scientific advancement are structurally short of core commodities from foods to fuels. They consider investing in these technologies an issue of national security.
More than anything, it’s compelling due to all the transformative technologies discussed in the next section that sit on offer waiting to be fully exploited.
Dozens of Nobel-Worthy Technologies Waiting to be Fully Exploited
Organism selection and engineering
Designing the Desired Molecules
For engineering of individual molecules like proteins or enzymes, directed evolution (DE) has been dramatically sped up by new high-throughput microfluidic systems, while decisions over next rounds can now be guided autonomously by AI.
But DE may have already been passed by AI models in efficiency and effectiveness. PLMs, AF2, NNPs, etc. help to rapidly explore the evolutionary landscape and generate candidates de novo according to pre-specified conditions. We’ve even seen startups leaning fully into the in silico experimentation loop, spinning up massively parallelized NNP simulations of enzymes and running DE entirely within computers.
Picking the Right Organism
To figure out how to most efficiently produce the desired molecule, researchers are increasingly looking outside of just E. coli and yeast. An interesting trend has slowly emerged where researchers prospect across the tree of life for organisms that natively express the closest genetic pathway to the one they ultimately desire to engineer and slightly tweak that instead of massively overhauling the highly studied model organisms.
For example, LBNL researchers recently made a handful of CRISPR edits to a fungi such that it expressed heme and a key antioxidant at the levels of commercial plant-based products like Impossible but without the expensive post-processing – the grown organism is the product.
Making this the norm will require plenty more basic science (e.g. better plant transfection tech, GRN & metabolic models) as we still have shockingly poor understanding of non-model organisms (e.g. only last year did a complete genome become available for sugarcane – the most common cash crop). The FRO Cultivarium is dedicated to building standardized, open source toolkits for as many non-model organisms as possible.
As seen below, using unconventional organisms isn’t quite as uncommon as one might think. Fun fact: eggs have been the dominant bioreactor for flu vaccine production for 70 years and monoclonal antibody manufacturing only took off once the field found organism-market-fit when people stumbled upon Chinese Ovary Hamster cells.
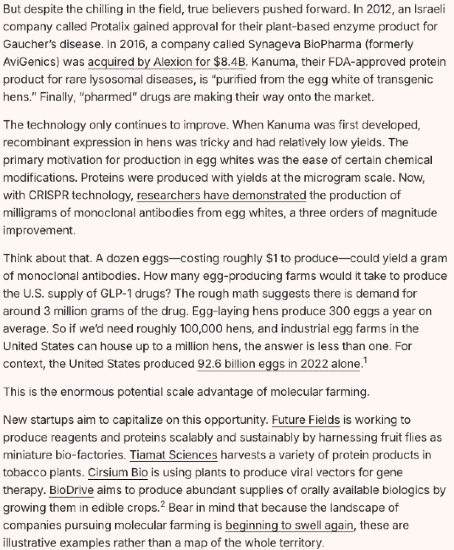
Engineering the Organism
Once the organism is selected, its internal circuitry must be reengineered to create an assembly line of enzymes and reactions that pumps out the desired end product. Though that engineering process is still far from being productized, we’ve come a long way in the last two decades in our ability to make model organisms make what we desire. For instance, LBNL researchers engineered yeast to produce vaccine adjuvant QS-21 by transplanting a whopping 38 different genes from six organisms – building one of the longest biosynthetic pathways ever.
Once the design of the biosynthetic pathway matures, researchers can maximize the engineered organisms’ downstream efficiency & productivity at manufacturing scale by manipulating its metabolic circuits with new metabolic simulators that combine modern AI with mathematical models and metabolic atlases built over many decades.
Some groups are taking moonshot-esque approaches to try to get around traditional growth-production metabolic tradeoffs. One introduced new-to-nature redox factors that are functionally orthogonal to the canonical NAD+ and NADP+ so as to potentially get around traditional pathways that drive catabolism and anabolism in opposite directions. Other groups are working on artificially speeding up the division of microbes by identifying the minimum viable genome.
Even getting individual organisms’ pathways optimized isn’t enough. When placed in large-scale bioreactors, they evolve over time away from producing the target molecule to prioritize their own growth. Ultimately, as few as 15% of cells actually produce the desired output and the rest are freeloaders, consuming valuable feedstocks without making anything useful.
To realize stable, high-yielding and predictable cell factories at scale, researchers are now engineering circuitry across all scales: from the initial circuit design to single-cell productivity optimization to entire populations. It’s like a more rudimentary version of compute now being developed from individual transistors all the way through to entire datacenters as a unified system.
Enduro developed genetic biosensors that tightly couple signals of desired product presence with cell survival. Essential genes are upregulated when the cell produces and senses the target substance and downregulated when they don’t, effectively making the cell pathologically addicted to producing the desired substance. They claim >30% titer and 5x fermentation time improvements. Meanwhile, extracellular or population-wide circuits like temperature or quorum-sensing were developed by teams like Evonik. Future pathways may integrate numerous signals.
Implementing Those Designs
Recent advances in genome-editing technologies enable directed, multiplexed, genome-wide engineering in a growing number of relevant microorganisms. Moreover, these highly multiplexed gene editing assays can be used to test the predictions of the AI models referenced above and generate training data at ever-greater scales.
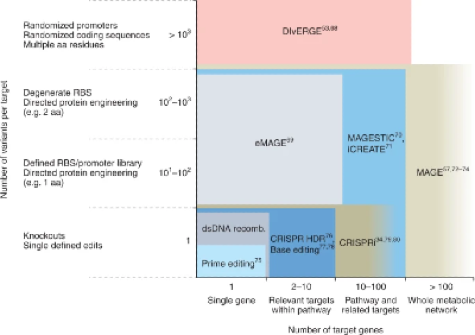
Picking the Right Cell Media to Grow the Organisms
Cell-culture media is the #1 need for upstream innovation, per an industry survey. Growth factors, recombinant proteins, etc. are all based on pharmaceutical-grade ingredients that can cost as much as millions of dollars per gram. Biomanufacturing anything other than high-value patented drugs will require orders of magnitude cheaper ingredients. Startups are working on developing and manufacturing cheap alternatives, AI-based predictions of what ingredients to use with a given organism, and even using waste products as a feedstock to cut input costs to $0. Meanwhile, Prolific is trying to utilize optogenetics’ light-based control over genetic circuits to avoid the need for such expensive molecules entirely.
Cell-Free
An approach that does away with living organisms entirely, cell-free extracts from lysated cells only the internal ingredients essential for product synthesis (generally just the enzymes). It thereby does away with the overhead and complications of living cells.
Because enzymes on their own work ~100x faster than whole cells, this could theoretically increase productivity far beyond the 3-4 titers per hour per liter that biomanufacturing has seemingly topped out at. Moreover, the field is boosted by the parallel development of improving enzyme immobilization technologies as well as AI models for predicting enzymes that can survive better in such environments.
Manufacturing Techniques & Bioreactor Design
The pharma industry started with large stainless steel batch-fed tanks and with its conservatism has been slow to try anything else. Biomanufacturing for other industries began by porting over pharma’s setup and only somewhat recently has the field re-evaluated from the ground up how to best design bioreactors for their cheaper markets and their particular products. It feels like a teenager questioning everything its parents told them and forming their own views – and we’re here for it.
We’re excited about the rise of technologies that allow similar yields with a lower upfront capex and smaller footprint and those that allow faster iteration cycles with more information gleaned per cycle.
Following the long secular rise in demand for single-use bioreactors within pharma, some industrial fermenting startups have also recently rotated towards smaller, modular reactor designs to scale out not up, betting on learnings from rapid iteration more than economies of scale.
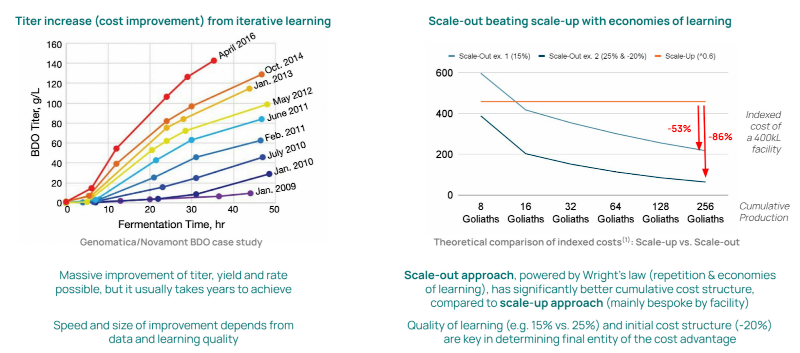
The next major trend within both pharma and industrial biomanufacturing is continuous manufacturing, with companies claiming 15-50% cost reduction. This approach’s major risk is contamination, so a startup is pursuing UV-sterilized bioreactors.
There are also product-specific techniques worth paying attention to. Cell-free is being applied to simple products like chemicals that don’t need the elaborate pathways that cells provide and to products that would be toxic to cells. For instance, companies like Solugen are using enzymatic and metal catalysis to combine the strengths of both chemicals and biology to produce chemicals at scale. Meanwhile, the food sector has its own laundry list of tech to develop. And, the pharmaceutical industry is using microfluidic systems for mRNA & cell therapy production, cell-free for products like ADCs with toxic byproducts, in-vivo cell expansion, acoustophoresis to suspend mammalian cells in media without physical contact for higher density, etc.
Scaling to Larger Reactors
Startups focused on making the scaling up from small to large bioreactors as seamless and predictable as possible are developing digital twins, simulation, identifying scale-independent data & models, 3D printing for physically modeling mimicking large scale at smallest, etc.
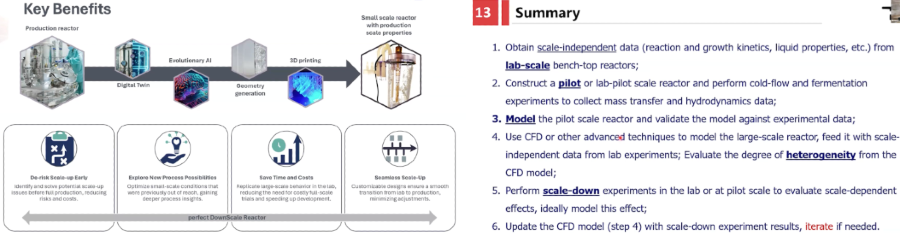
Optimal Conditions At Scale
Not surprisingly, a major trend is the integration of real-time monitoring of growth conditions that enable large datasets for AI analysis and automated closed-loop adjustments. While Raman spectroscopy is the main sensing technology used today, novel methods like biosensors and eventually possibly quantum sensing will provide single-molecule specificity.
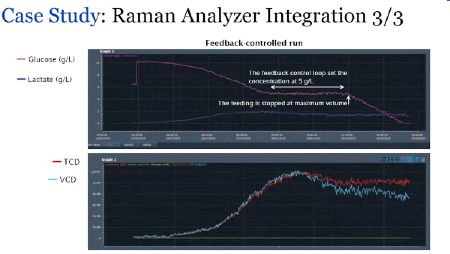
Towards Maximum Ambition
So far no team has dared to combine more than a couple of the 40+ innovative technologies I’ve detailed above.
Though we’d love to be proven wrong, these nascent industries likely can’t support one startup commercializing each individual technology. Each player faces the cold start dilemma, slow sales cycles, and their margin eats into or evaporates whatever efficiency gains their tech provided in the first place. Instead, these markets likely necessitate a SpaceX-level of vertical integration and control over each step in the process to ensure maximal efficiency.
Moreover, the more a company handles in house, the more it can train its models to predict which combination of changes will be best (e.g. x strain edited in y way with z growth factor). This process of product development from organism selection to optimal conditions is a problem of combinatorial explosion and thus inherently well suited to AI. The lock-in and data flywheel this creates helps transition these businesses away from being purely linear scale ups of capital intensive manufacturing processes relying strictly on basic economies of scale (which are unusually hard to achieve in biomanufacturing due to contamination, etc. Indeed the average size of the world's largest bioreactors has fallen by 20%+ in the last 8 years).
While we don’t have strong views on which technologies to combine for each given product, the intuitive starting point is those that are both the most well-understood or productized that also have the biggest impact on ultimate yields. The minimum is likely some bioreactor innovation (e.g. continuous, sterile) plus real-time monitoring and automation plus several advanced biological solutions like multifaceted organism engineering. After early-stage rounds, startups may continue sequencing in additional technologies.
Maybe synthetic biology is still simply too early with each technology listed above not sufficiently productized to be readily integrated into a well-oiled manufacturing platform. But that’s a risk we are prepared to take and are excited that some teams have started to take note. A new biomanufacturing startup is the first to combine three technologies at once while one of the largest scaled alt-meat startups embraces CRISPR-engineered cell lines being necessary for their survival in addition to full-stack redesign of its manufacturing process.
Whoever figures this out has access to massive markets, like the following:
- Science-driven consumer, which has long been a focus of Compound, remains largely untapped. We believe more than ever that the best first-order markets are vanity products (hair and skin health, weight-loss, muscle growth) and consequence-free indulgence (e.g. curing hangovers, sugarless sweetness). These businesses must mix science innovation with the viralness of Poppi marketing and artistic branding. We’re also interested in other solutions to the problems of both consumers (e.g. pitless cherries, romain lettuce with kale nutrition) and producers (e.g. non-browning bananas). While some are concerned about consumer’s hesitancy towards GMO products, we firmly believe that vague fear is overwhelmed by products that actually address their problems. For instance, the introduction of baby carrots and seedless snack-sized oranges increased total US carrot and citrus consumption by 30% in the first year alone.
- Biosimilars are finally starting to gain meaningful adoption, got major regulatory breaks in the last two years (e.g. possible waiving of Ph3 trials and eliminating switching studies), and over the next decade 118 biologics representing in $232B market value will lose patent protection. Yet, the opportunity remains wide open as only a dozen molecules with patents expiring have biosimilars in development. If the development of non-novel drugs continues to become ever-more commoditized, the best place to be in that market may well be a highly efficient manufacturer.
- A gray market for semi-legal “research use only” peptides (but that people use in a DIY fashion) has quietly spun up – we’ve anecdotally heard that some producers have scaled to $100M in revenue. The market needs a highly trust-worthy, respectable manufacturer that can also run human safety and efficacy trials as many peptides lack any rigorous benchmarking.
- Biologics more broadly show no sign of slowing & are getting more complex to manufacture each year, offering an opening for techniques that dramatically simplify the process. For instance, maybe cell free or synthetic cells could be used to manufacture fully personalized cancer vaccines in a distributed, cost-effective way.
- Biomanufacturing of essential active pharmaceutical ingredients, now recognized as a national security issue
- Entirely novel, high performance products like spider silk or materials with self-healing properties can garner high-end price points from defense, aerospace, or semis like analogous materials of the past from teflon to bulletproof glass to now superalloys for hypersonics. Some such materials like velcro are able to slowly make it to mass consumer markets.
- The ceaseless march of climate change means that all commodities must be rebuilt from scratch to be green at some point. These are trillion dollar markets but should be tackled only once a vertically integrated approach has been perfected enough to be cheaper than incumbent techniques as a green premium can’t be relied upon in many markets, especially for consumer-oriented products.
We at Compound continue to believe that synthetic biology and biomanufacturing are only a matter of time, despite mass pessimism. If you’re taking an integrated, maximally ambitious approach to tackling the markets above or ones we haven’t thought of, please reach out!